In complex and precise industrial system architectures, Safety Integrity (SI) serves as the cornerstone for safeguarding personnel, assets, and the environment. The concept of “failure” within this context is a critical node that demands comprehensive and in-depth understanding. Accurate identification and detailed classification of failures not only facilitate the stable operation of systems but also strengthen the essential safety barriers.
1. What is Failure?
From a professional perspective, failure within the domain of safety integrity refers to the inability of safety-related systems, subsystems, or individual devices to perform their intended safety functions. This loss of capability is not an isolated incident; it disrupts the pre-established safety balance of the system, increasing the likelihood of potential risks evolving into actual hazards. For example, in a petrochemical refinery, if the gas leak detection system fails to trigger an alarm during a minor combustible gas leak, the entire facility is exposed to the risk of explosion and fire.
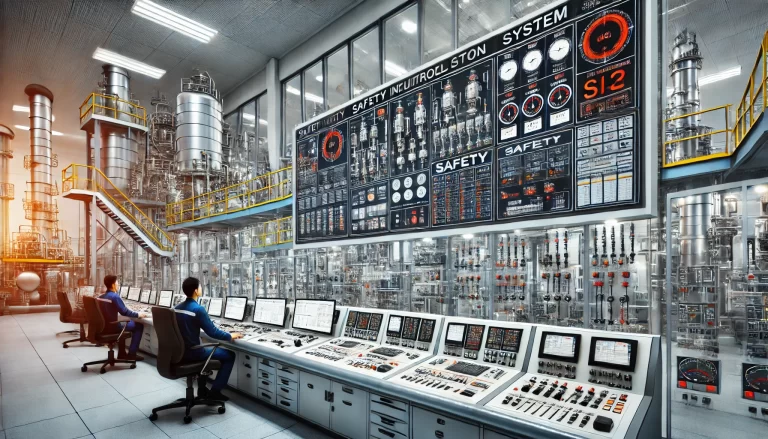
2. Categories of Failures
Failures in safety integrity levels are typically categorized into six types:
2.1 Early Failures
Early failures occur predominantly during the initial phase of equipment or system operation. They often stem from theoretical flaws in design, manufacturing defects, or improper handling during installation and commissioning. For instance, a newly designed automated production line’s safety light curtain may malfunction under strong light interference due to inadequate consideration during design, compromising its protective function. These failures tend to decrease rapidly over time as the system moves past the initial “break-in” period, with problems typically being systemic or holistic in nature.
2.2 Random Failures
Random failures are unpredictable and are not influenced by operational time or usage frequency. They result from unforeseeable accidental factors and follow probabilistic distribution, making it difficult to predict the exact occurrence. However, with extensive sample data and probabilistic models, their overall likelihood can be estimated, informing redundancy design strategies.
2.3 Wear-Out Failures
Wear-out failures occur as components deteriorate over extended periods due to continuous wear, fatigue, and corrosion. For example, turbine blades in a thermal power plant are subjected to high-temperature and high-pressure steam, causing material creep, thinning, and strength reduction over time. This degradation leads to increased vibrations and compromised operational stability, ultimately affecting system safety. Such failures are closely related to service time, with a higher probability of occurrence as usage prolongs, often accompanied by progressive performance decline (e.g., increased noise and energy consumption).
2.4 Systemic Failures
Systemic failures are caused by conceptual deviations in system design, software algorithm errors, or procedural loopholes. For instance, if a chemical process control system’s software contains logical flaws that miscalculate reaction parameters under specific raw material ratios, it may issue erroneous commands, leading to uncontrolled reactor temperatures. These failures are not random; they consistently reoccur under specific conditions and are common across identical systems. Addressing these failures requires redesigning the system and overhauling operational procedures.
2.5 Dangerous Failures
Dangerous failures compromise critical safety defenses and often result from sudden damage to key protective components or safety circuits. For example, if a mining hoist’s braking system experiences mechanical fatigue and a critical brake pad fractures, the elevator could lose its braking capability and fall rapidly down the shaft. These failures have severe consequences, leaving personnel and assets highly vulnerable, with virtually zero tolerance for error. Therefore, rigorous preventive and monitoring measures are imperative.
2.6 Safe Failures
Safe failures are protective actions deliberately triggered by equipment to ensure safety. For example, when a car engine overheats, the onboard computer may cut off the fuel supply to prevent engine damage. Although this action results in temporary downtime, it prevents catastrophic outcomes. This type of failure acts as a safety fallback mechanism, requiring precise calibration of trigger thresholds to avoid excessive protection that could hinder operational efficiency.
3. Tailored Mitigation Strategies for Different Failure Types
Each failure type is akin to a distinct “illness” requiring customized “treatment plans”:
Early Failures: Call for stricter design and manufacturing review processes.
Random Failures: Justify the construction of redundancy architectures and probabilistic safety models.
Wear-Out Failures: Emphasize the importance of preventive maintenance programs.
Systemic Failures: Demand innovative design thinking and comprehensive process audits.
Dangerous Failures: Necessitate multi-layered emergency braking and monitoring systems.
Safe Failures: Require proper calibration to balance protection and productivity.
In safety integrity level (SIL) assessments, precise failure classification is the foundation for quantifying risks and matching appropriate protective measures. This comprehensive approach ensures robust and resilient industrial systems that prioritize safety without compromising efficiency.